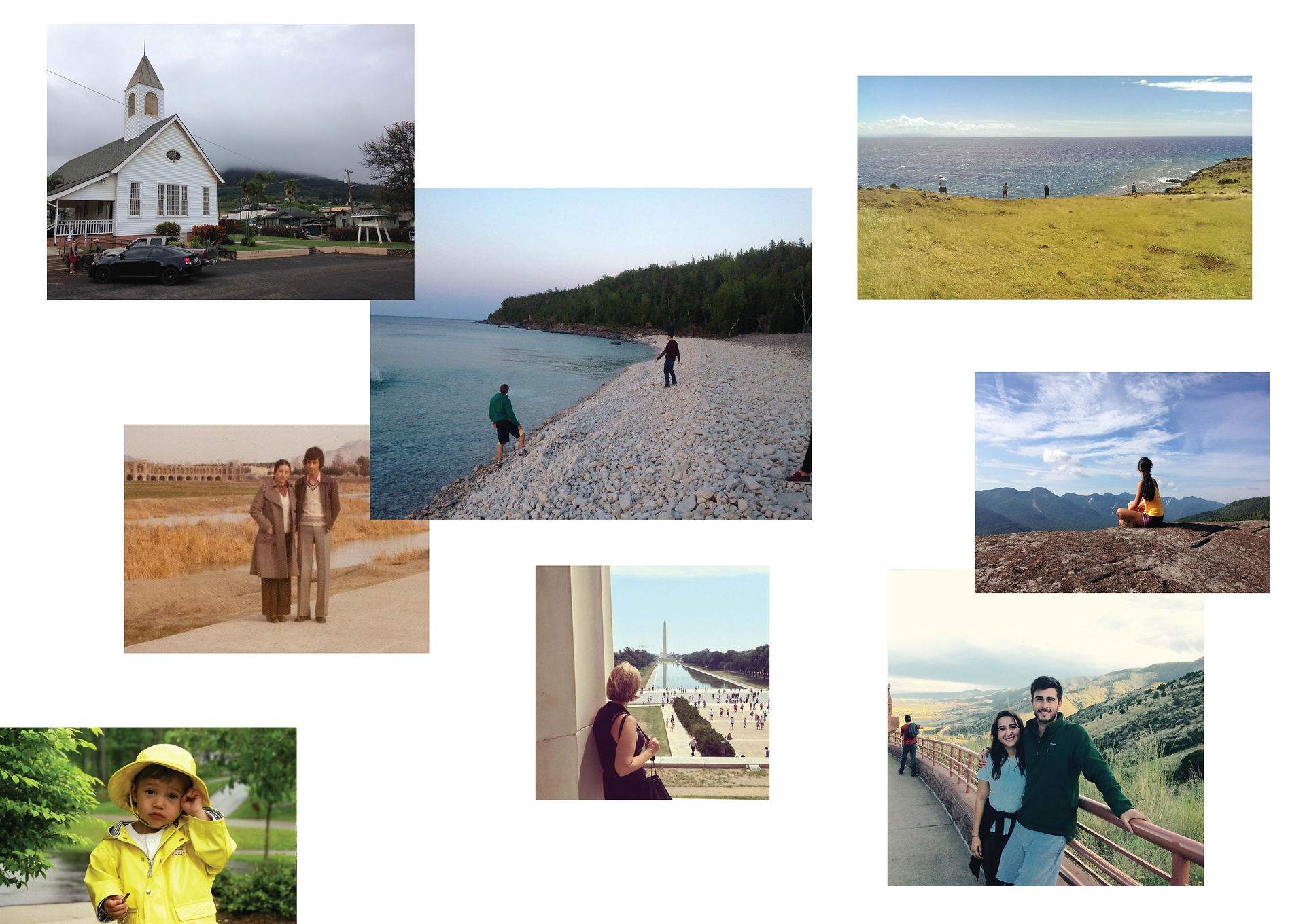
Cameron Fattahi
Writing Portfolio
Converging Dendritic Spine Pathology in Neuropsyciatric Disorder
For the requirements of my minor, I needed to take an Upper Level Writing Course in my major. I opted to take a self-directed, advanced reading course, Psychology 420, in which I read a large number of research papers and compiled a literature review. I chose to explore and summarize the scientific literature on shared neural abnormalities that underlie a few notable neuropsychiatric disorders (autism, fragile-X syndrome, Alzheimer’s disease, and schizophrenia).
The essay was, surprisingly, wildly fun for me. Part of the fun was that I was able to do a bit of research on psychiatric disorders in general and the evolution of knowledge that scientists have accumulated. Given the rhetorical situation, I think the review works very well. It is segmented, just as a journal review would be, with an abstract, introduction, et cetera, and is written in a tone that is understandable and (potentially) engaging to any reader—not just those in the science community. For these reasons, it excels. While it is difficult (and often inappropriate) to incorporate personal narrative into a scientific review, I was able to add a bit of my own personality by connecting the modern understanding of these disorders to a historical knowledge—a relatively unique undertaking within the scientific lit-review world. In the future (if and when I write other literature reviews), I will likely follow a similar format but work to vary sentence structure within the boring middle part of the paper. I will also work to provide more informative and summative conclusions, though mine, here, are adequate.
Abstract
Neuropsychiatric disorders, including autism spectrum disorder, fragile-X syndrome, schizophrenia, and Alzheimer’s disease, are caused by impairments in the precise wiring of neural circuitry and brain volume abnormalities. Of specific interest in these networks are dendritic spines, which form during dendritic morphogenesis and create excitatory synapses with neighboring neurons, allowing for precise communication. Abnormalities in spine number and dysfunctions in spine plasticity are associated with impairments in proper cognitive functioning. Though each neuropsychiatric disorder is unique in its clinical symptoms and manifestations, it is shown that dendritic spine pathology suggests a converging underlying principle, contributing to the elucidation of the neuropathological mechanisms of psychiatric disease. Future research should continue to elucidate these converging pathways and therapies should target their impairments.
Introduction
Neuropsychiatry is a discipline of medical study that seeks to understand the neurological bases of psychiatric disorders. Though the intertwined relationships have only begun to reach true manifestation within the past century, the implications have been suggested since the beginning of psychiatric study. In 1812, Benjamin Rush, the now-accredited “Father of American Psychiatry,” made the following statement in a letter to former President, John Adams: “I have endeavored to bring [diseases of the mind] down to the level of all other diseases of the human body, and to show that the mind and body are moved by the same causes and subject to the same laws,” (Martin, 2002). Recently, with the advent of modern technologies, including MRI and genetic analyses, researchers have been able to show that these “same laws” do in fact underlie many aspects of psychiatric disease, and the neurological bases of such disorders are beginning to reach fruition.
Psychiatric diseases, including autism spectrum disorders (ASDs), fragile-X syndrome, schizophrenia, and Alzheimer’s disease are the result of impaired brain functioning. As part of the central nervous system, the brain contains neurons, electrically and chemically excitable cells that allow for information to be relayed throughout the brain and the rest of the body. Such signaling is what comprises cognition and other neurological functioning. Neurons form a dense network in the brain that maximizes signal conduction and processing. One neuron meets another at a junction called the synapse. It is here that an incoming neuron (the presynaptic neuron) passes its signal to another (the postsynaptic neuron). More specifically, the signal leaves the presynaptic neuron from the axon (the output end of the neuron) and enters the postsynaptic neuron at the dendrite (the receiving, or input, end of the neuron). A very precise assembly is needed for normal brain functioning, and any faults in the wiring may contribute to disorder (Penzes, 2011).
Neurons grow and assemble in the fetal process of neurogenesis, in which neurons navigate their way into the dense neural network and form synapses with specific neurons in the process of synaptogenesis. In synaptogenesis, dendrites of the postsynaptic neuron grow outward to meet with axons of the presynaptic neuron. The specific outgrowth of dendrites during development is termed dendrite morphogenesis (Kulkarni, 2012). Two key processes occur during dendrite morphogenesis: development of the dendritic arbor and development of dendritic spines. The dendritic arbor is the vast tree-like network of dendrites that extends from the soma, or cell body, of each neuron. It is a complex network that is constantly reshaped and reconfigured in response to presynaptic messages and postsynaptic control (Kulkarni, 2012). Covering the shaft of individual dendrites in high-functioning regions of the brain are small, knobby protrusions called dendritic spines that individually meet with a corresponding axon bud, forming an excitatory synapse. In dendritic morphogenesis, dendritic spines grow from primordial structures called filopodia, which actively seek out axonal buds, form synapses, and develop into mature spines (Fiala, 2002). The density of spines in the dendritic arbor is said to directly correlate with the level of excitability of a neuron (Fiala, 2002). Therefore, an increased dendritic spine density from normal suggests over-excitability and a decreased dendritic spine density suggests lowered excitability.
But the assembly and growth of spines during brain development is not the only stage of importance. There is also a pruning stage whereby spines are eliminated from the dendrites. Typically, dendrite morphogenesis occurs during fetal development and childhood, increasing spine density before and after birth. As a child begins adolescence, pruning is initiated and the dendritic spine number is reduced over time to a steady number that lasts most of adulthood (Penzes, 2011).
Dendritic spines are also regulated throughout one’s lifetime in the process of synaptic plasticity. Though genetics plays a role in the development of neural networks, much of the formation and further plasticity is directly dependent on experience (Blanpied, 2004). Spines themselves are dynamic structures (Kasai, 2010), and each spine can be thought of as its own “computational unit” of the brain because it undergoes specific biochemical regulation, controlling its own plasticity and morphology (Blanpied, 2004). Dendritic spines contain vast amounts of actin, a protein that mediates cytoskeletal structure, allowing a spine to alter its morphology, enabling plasticity in response to specific stimuli. Long-term potentiation, a well understood process whereby frequently used synaptic pathways are strengthened and dendritic spines are enlarged (Kasai, 2010), is one such example of spine dynamics. Synaptic plasticity plays an important role in learning and memory (Colvin and Kwan, 2014).
Recently, scientists have begun to focus on dendritic impairments as neurobiological causes of psychiatric disease, bringing to reality the endeavors of Benjamin Rush. Many researchers have found that disruptions in dendrite morphology, whether in the dendritic arbor or in spines and synapses, underlie pathologies in many neuropsychiatric disorders. More specifically, alteration in spine number, size, shape, and ability to undergo activity-dependent change has also been correlated with disorder (Fiala, 2002). Interestingly, the morphological disruptions found in many neuropsychiatric disorders are time-dependent, often corresponding with disorder age of onset and presented clinical symptoms (Penzes, 2011).
Of particular interest to dendritic spine pathology studies are those disorders that feature clear disruptions in cognition and the ability to process information, as they would suggest marked deficiencies in brain functioning. Such disorders include autism spectrum disorders, fragile-X syndrome, schizophrenia, and Alzheimer’s disease, each unique in its clinical symptoms, but featuring similar underlying dendritic spine pathologies. We will look at each specific disorder, noting its psychological understanding, clinical presentation, and suggested dendritic pathology, ultimately aiming to reveal a converging mechanistic impairment that contributes to the general brain dysfunction observed in neuropsychiatric disorders.
Autism Spectrum Disorders
Autism spectrum disorders are a group of similar behavioral syndromes affecting roughly 1 – 2.6% of children globally (Hansen, 2015), with initial juvenile presentation typically occurring around the age of 2 or 3. The disorder is clinically defined (Sparks, 2002), featuring deficits in social and emotional interaction, impaired language use and comprehension, and repetitive behaviors. MRIs and postmortem studies have discovered brain abnormalities, particularly in limbic areas including the hippocampus, amygdala, and cerebellum (Sparks, 2002). More specifically, these areas of the brain, in addition to the cerebrum, were found to have notably greater volumes in ASD children than in normally-developed children (Sparks, 2002). Such knowledge contributes to the understanding of the observed clinical symptoms, as the cerebrum controls cognitive functioning, the limbic system controls emotion and behavior, and the cerebellum controls precise motor movements.
More recent research has lent support to this same discovery by elucidating some of the impairments in dendritic spine morphogenesis underlying the disorder. Postmortem studies using ASD brains have shown an increase in spine number of cortical pyramidal dendrites in the frontal, temporal, and parietal lobes of the brain (Penzes, 2011), and decreased dendritic arborization in specific areas of the hippocampus (Kulkarni, 2012). It is postulated that in ASD patients, dendritic spine formation during fetal growth and early childhood is over-activated, creating too many spines. Additionally, the pruning of spines is thought to be dramatically reduced at adolescence, creating an excess of dendritic spines throughout a patient’s lifetime (Penzes, 2011). Such beliefs form the basis of the suggested hypothesis that ASD individuals have hyperactivity in short-range circuits in the brain, but exhibit hypoactivity in longer-ranged circuits that connect different regions of the brain together.
On a more mechanistic level, cell-adhesion molecules, molecules expressed in axons and dendrites during synaptogenesis, play a key role in the regulation of synaptic development, and are often mutated in ASD (Toro, 2010). These molecules have been found to contribute to the activity-dependent synaptic reorganization of neural networks in the brain. Additionally, scaffolding proteins that maintain integrity and morphological plasticity of individual dendritic spines, are found to be impaired in ASD (Toro, 2010). As both cell-adhesion molecules and scaffolding proteins facilitate the assembly of specific synapses and control homeostatic dendritic spine dynamics, synapse dysfunction is a notable associated pathology underlying ASD, and may lead to many of the observed cognitive abnormalities.
In summary, ASD patients have shown increased total dendritic spine number, impaired synaptic plasticity, and a pathologically increased volume in cerebrum and limbic regions of the brain. Molecules active in synaptic plasticity models are also disrupted. These impairments directly correlate with the clinical symptoms present in ASD patients.
Fragile-X Syndrome
Much like ASD, fragile-X syndrome is a neuropsychiatric disorder that features impaired cognition, social behavior, and language deficits, in addition to aggression and inability to maintain attention. It is the leading inheritable cause of intellectual disability. Research has shown that fragile-X syndrome pathology is associated with impaired synaptic plasticity (Kwan, 2012). Specifically, the mechanistic processes underlying both long-term potentiation and long-term depression, whereby synaptic pathways are increased in strength and decreased in strength, respectively, exhibit irregular functioning (Berry-Kravis, 2014). Similar to ASD, spine dysmorphogenesis has been implicated in fragile-X syndrome, whereby an increased number of spines on pyramidal neurons in the cerebral cortex has been observed (Penzes, 2011). Furthermore, these dendritic spines are specifically noted to be “long, thin, and immature” (Kulkarni, 2012), clearly suggesting morphological dysfunction.
As fragile-X syndrome is an inheritable disorder, elucidating its genetic causes is central to the complete neurological understanding of the disorder. The disorder results from the silencing of the fragile mental retardation 1 gene (Fmr1), a gene that codes for FMRP, an associated protein. FMRP is known to regulate production of the neuronal nitric oxide synthase 1 (NOS1) protein (Kwan, 2012). NOS1 is an important protein in the development of neural circuitry because it produces nitric oxide (NO), a molecule known to function in nervous system development and synaptic plasticity (Bear, 2004). Both FMRP and NOS1 protein are concurrently expressed in the synaptogenesis of pyramidal neurons in the frontoparietal operculum and anterior cingulate cortex, fetal brain regions that prospectively function in language development and comprehension, and social and behavioral functioning, respectively (Kwan, 2012). FMRP is non-functioning in fragile-X syndrome patients and NOS1 expression is dramatically reduced, suggesting a dysfunction correlated with the observed clinical symptoms. Furthermore, blockade of NOS1 functioning in animal models has revealed synapse dysgenesis and loss of dendritic spines (Colvin and Kwan, 2014).
Additionally, FMRP is associated with another protein, Rac1, which facilitates dendritic spine development and synapse formation (Kulkarni, 2012). Studies using Fmr1 knockout mice have shown hyperactivation of the Rac1 protein, suggesting a possible cause of the overproduction of dendritic spines found in pyramidal neurons of fragile-X syndrome patients, and hinting at the aberrant synaptic plasticity (Kulkarni, 2012). Therefore, the molecular and genetic bases of fragile-X syndrome are well underway in being explored, and suggest aberrant synaptic plasticity, an increase in overall dendritic spine number, and a physical dysfunction in dendritic spines, supporting the manifestation of clinical symptoms.
Schizophrenia
Schizophrenia is a neuropsychiatric disorder with diverse impairments in perception and cognition, affecting between 0.5 – 1.0% of people globally. It is more common in males, often presenting with a significantly increased degree of symptoms compared to females. Additionally, males with schizophrenia often see disorder onset at an earlier age than females, reduced response to treatment, and decreased overall outcome (Glausier, 2013).
Schizophrenic symptoms can be categorized into three groups: positive, negative, and cognitive. Hallucinations and delusions are included in the positive symptom category. Negative symptoms include impaired ability to express one’s emotions, inability to enjoy activities that were once pleasurable, speech impairments, and lack of motivation during goal-oriented tasks. Cognitive symptoms are those that are associated with the cerebrum, including impaired executive functioning, attention, and working memory capacity. Positive and negative symptoms are typically manifested in late adolescence and early adulthood, while cognitive impairments are prevalent very early on, even before diagnosis (Glausier, 2013).
The diverse range of symptoms present in schizophrenic patients suggests that a number of different brain regions are impaired. In general, researchers have found decreased gray matter throughout the cortical areas of the brain (Penzes, 2011). Gray matter is the portion of the central nervous system that contains neuronal cell bodies, dendrites, and unmyelinated axons. White matter contains only myelinated axon fibers. Additionally, this gray matter decrease is suggested to be progressive throughout the entire course of the disorder (Glausier, 2013), becoming worse and worse with time. However, postmortem studies have found that this decreased volume of gray matter is not directly from neuron loss, but rather from decreased pyramidal cell body size in the cortex and decreased dendritic arborization (Glausier, 2013). More specifically, pyramidal neurons in layer three of the dorsolateral prefrontal cortex — an area of the frontal lobe that controls executive functioning — show significantly decreased cell body sizes (Penzes, 2011). Concurrently, overall dendritic spine density is lower in layer three neurons of the dorsolateral prefrontal cortex in these patients than in normal individuals (Glausier, 2013). In addition, the primary auditory cortex shows similar spine impairment (Penzes, 2011), implicating some of the negative symptoms observed. Layer three neurons project to other areas in the cortex and to the thalamus, suggesting a broader range of impairment that may explain the numerous types of symptoms observed clinically. This impairment is postulated to be a result of excessive spine pruning during adolescence and early adulthood (Penzes, 2011), further correlating with and providing support for the symptoms associated with disorder.
Additional research has suggested that schizophrenia pathology results from impaired activity-dependent plasticity in dendritic spines of the cortex. Evidence comes from the observation that a specific molecule, phencyclidine, acts as an antagonist of NMDA receptors in schizophrenic brains, which are important receptors for synaptogenesis and pruning (Kasai, 2010). Agonists for the receptor and molecules blocking the action of phencyclidine have revealed a marked decrease in schizophrenic symptoms when used in patients.
Additional deficits are observed in brain regions outside of the cerebral cortex, including the striatum and hippocampus. The striatum is a subpart of the basal ganglia and acts to coordinate motivation with body movement. Interestingly, dendritic spine density was found to be increased in this area (Glausier, 2013), which is counterintuitive to the results of the cortex dendritic spine findings. However, the impaired striatal neurons project to another part of the basal ganglia where they act to inhibit specific functions. Therefore, the increased amount of inhibitory actions may cause some of the observed impairment, though researchers are unsure if this is a cause or effect of the disorder (Glausier, 2013). The hippocampus, a small part of the limbic region of brain controlling emotional expression and memory, is also impaired in schizophrenia. Unlike the striatal abnormalities in schizophrenic brains, but similar to those of the cerebrum, the hippocampus has a notably reduced volume of gray matter and a reduction in dendritic spine density (Penzes, 2011), therefore suggesting hypoactivity in the region of the brain underlying emotional control and memory.
Alzheimer’s Disease
Alzheimer’s disease is a neurodegenerative disease typically first observed in a patient at the age of 65. After diagnosis, memory loss increases with time, concurrent with deficits in intellectual reasoning and other cognitive functioning. Alzheimer’s disease has long been characterized by amyloid plaques, neurofibrillary tangles, and neuronal cell death, though researchers suggest that synaptic dysfunction is a preceding abnormality that instigates these distinguishing neural impairments (Penzes, 2011). Compared to ASD, fragile-X syndrome, and schizophrenia, Alzheimer’s disease has decades of research lending to its defined pathology. Using postmortem studies of Alzheimer’s brains, researchers have consistently found synapse dysfunction and decreased dendritic spines (Penzes, 2011), particularly in the cerebral cortex and hippocampus. Spine dysgenesis is thought to precede the formation of neurofibrillary tangles and amyloid plaques because it is evident in mild cognitive impairment, a precursor disorder to Alzheimer’s disease (Penzes, 2011). Unlike spine dysgenesis, neurofibrillary tangles and amyloid plaques are only present in full Alzheimer’s development. Furthermore, spine impairment is significantly increased in full Alzheimer’s compared to mild cognitive impairment, suggesting that spine dysgenesis increases with disease progression. Researchers have determined that the dendritic spine density decreases are central to the pathology of Alzheimer’s rather than a byproduct of disease because the spine impairment observed is often much greater than what would be expected purely from neuronal cell death (Penzes, 2011).
Molecularly, the Rho branch of proteins (similar to Rac proteins being investigated in fragile-X syndrome pathology) is found to regulate synapse formation and spine maintenance (Kulkarni, 2012). Using mice models for Alzheimer’s disease, researchers found an increased Rho activity compared to non-Alzheimer’s mice. It has been suggested that increased Rho activity causes shortening of dendritic spines and synapse reduction, adding to the understanding of Alzheimer’s disease pathology.
Therapeutic Possibilities
The finding that dendritic spine pathology and synaptic dysgenesis are associated with a number of neuropsychiatric disorders is very important for therapeutic intervention, and provides a bright outlook for afflicted patients. It is again important to note that dendritic spines are dynamic structures and change morphologically over the course of human development. As supported by the literature, the dynamic abilities of dendritic spines suggest that they can be specifically modified by the actions of molecules. Many different genes code for the production of proteins that play key roles in the morphogenesis of spines and corresponding synaptogenesis (Penzes, 2011). Thus far, a number of protein impairments have been found in neuropsychiatric disorders, including: cell-adhesion molecules and scaffolding proteins in ASD, FMRP in fragile-X syndrome, phencyclidine in schizophrenia, and Rho proteins in Alzheimer’s disease. However, these examples barely scratch the surface of elucidating the complete molecular control of dendritic spines. Continued exploration of the underlying genetic and molecular mechanisms involved in general dendritic spine morphogenesis and plasticity and that are impaired in specific neuropsychiatric disorders, will allow for careful therapeutic intervention aimed at regulating the disrupted molecular mechanism or gene. Because all neuropsychiatric disorders show generalized cognitive impairment and dendritic spine abnormality, it is thought that phenotypically converging mechanisms underlie the cognitive aspect of the disorders, and may be treatable with a single form of therapy (Colvin and Kwan, 2014).
One noteworthy example is the mGluR pathway most studied in relation to fragile-X syndrome (Bear, 2004). mGluRs, or metabotropic glutamate receptors, typically act to stimulate long-term depression, which as noted above decreases the strength of underused synaptic pathways. FMRP acts to stop long-term depression from over-occurring. In fragile-X syndrome, where FMRP is deficient, long-term depression cannot be stopped and mGluR activity becomes pathologically excessive, leading to dysfunction. Therefore, mGluR antagonists have been suggested as a possible way to combat excessive long-term potentiation, serving as a potential treatment option for fragile-X syndrome (Bear, 2004).
Recent studies have shown that FMRP and nitric oxide action in synaptogenesis are also found in ASD and schizophrenic brains (Colvin and Kwan, 2014), though not necessarily impaired. Nonetheless, the functionally convergent pathway implies the potential use of mGluR antagonists in other disorders, and moreover provides a concrete example of the extent to which a single therapeutic agent may serve many neuropsychiatric disorders by acting on common pathways.
Concluding Remarks
Normal functioning of the brain is dependent on a precise circuitry of neurons. As observed in neuropsychiatric disorders including autism spectrum disorder, fragile-X syndrome, schizophrenia, and Alzheimer’s disease, disruptions in the precise connectivity leads to disorder. Research has found varied abnormal spine densities across neuropsychiatric disorders, and synaptic plasticity is often impaired. Disruption may occur in early fetal development or in adolescent stages of pruning and onward. Time-dependent impairments may relate to the specific age of onset often characteristic of the different disorders. Though each disorder presents with varied clinical symptoms, they all show phenotypic convergence in impaired cognitive functioning. Such convergence suggests similar mechanistic pathways underlying the abnormalities observed in the dendritic spines of neuropsychiatric disorder patients.
Future research should work to elucidate these mechanistic pathways. Specific focus should be given to those that are similar across disorders, as they will suggest generalized methods for how to treat common symptoms of neuropsychiatric disorder.
References
Bear, M. F. (2004-07). The mGluR theory of fragile X mental retardation. Trends in neurosciences (Regular ed.), 27(7), 370-377.doi:10.1016/j.tins.2004.04.009
Berry-Kravis, E. (2014-04). Mechanism-based treatments in neurodevelopmental disorders: fragile X syndrome. Pediatric neurology, 50(4), 297-302.doi:10.1016/j.pediatrneurol.2013.12.001
Blanpied, T. A. (2004-06-15). Microanatomy of dendritic spines: emerging principles of synaptic pathology in psychiatric and neurological disease. Biological psychiatry (1969), 55(12), 1121-1127.doi:10.1016/j.biopsych.2003.10.006
Colvin, S. M., & Kwan, K. Y. (2014). Dysregulated nitric oxide signaling as a candidate mechanism of fragile X syndrome and other neuropsychiatric disorders. Frontiers in Genetics, 5, 239. doi:10.3389/fgene.2014.00239
Fiala, J. C. (2002-06). Dendritic spine pathology: cause or consequence of neurological disorders?. Brain research. Brain research reviews, 39(1), 29-54.
Glausier, J. R. (2013-10-22). Dendritic spine pathology in schizophrenia. Neuroscience, 251, 90-107.doi:10.1016/j.neuroscience.2012.04.044
Hansen, S. N. (2015-01). Explaining the increase in the prevalence of autism spectrum disorders: the proportion attributable to changes in reporting practices. JAMA pediatrics, 169(1), 56-62.doi:10.1001/jamapediatrics.2014.1893
Kasai, H. (2010-03). Structural dynamics of dendritic spines in memory and cognition. Trends in neurosciences (Regular ed.), 33(3), 121-129.doi:10.1016/j.tins.2010.01.001
Kulkarni, V. A. (2012-05). The dendritic tree and brain disorders. Molecular and cellular neurosciences, 50(1), 10-20.doi:10.1016/j.mcn.2012.03.005
Kwan, K. Y. (2012-05-11). Species-dependent posttranscriptional regulation of NOS1 by FMRP in the developing cerebral cortex. Cell (Cambridge), 149(4), 899-911.doi:10.1016/j.cell.2012.02.060
Martin, J. B. (2002-05). The integration of neurology, psychiatry, and neuroscience in the 21st century. The American journal of psychiatry, 159(5), 695-704.
Penzes, P. (2011-03). Dendritic spine pathology in neuropsychiatric disorders. Nature neuroscience, 14(3), 285-293.doi:10.1038/nn.2741
Sparks, B. F. (2002-07-23). Brain structural abnormalities in young children with autism spectrum disorder. Neurology, 59(2), 184-192.
Toro, R. (2010-08). Key role for gene dosage and synaptic homeostasis in autism spectrum disorders. Trends in genetics, 26(8), 363-372.doi:10.1016/j.tig.2010.05.007